Previous Research Activity
Motivation
In quantum optics, light is understood to exhibit both wave-like and particle-like properties. My research focuses on exploring the relationship between these two fundamental aspects of light.
Within the framework of quantum optics (quantum information processing), I have conducted research using three distinct approaches: theory, bulk optical experiments, and integrated photonic circuit experiments. At Hiroshima University (PhD program), I conducted theoretical research on quantum measurement. At Hokkaido University (postdoctoral researcher), I carried out experimental research on quantum measurement using bulk optical systems. At the University of Bristol, UK (postdoctoral researcher), I conducted experimental research on quantum silicon photonics using photonic integrated circuits.
Generation of macroscopic quantum superposition states
In this study, we theoretically analyzed the quantum interference between laser light and squeezed light from a photon-based perspective and discovered that it is possible to generate a macroscopic quantum superposition state.
Macroscopic quantum superposition states have been widely studied across various fields, ranging from fundamental research to applications such as quantum measurement with sensitivity beyond classical limits and the investigation of quantum entanglement in many-particle systems. However, until now, no scalable experimental method for generating macroscopic quantum superposition states has been established.
In quantum theory, light can be treated using two main approaches: one that considers it as a field (continuous variables) and another that treats it as particles (discrete variables). Until now, the interference effects between laser light and squeezed light have primarily been analyzed using the continuous-variable approach. In this study, we investigated these interference effects from a photon-based perspective, treating light as discrete variables. We discovered that a macroscopic quantum superposition state can be generated when the intensities of the two light sources are equal.
This result was experimentally demonstrated using five photons by a research group in Israel (Science 328, 879–881) and was featured in Science (Science 328, 835–836).
[1] PHYSICAL REVIEW A 76(3) 031806 (2007) [2] JOURNAL OF PHYSICS B 41(9) (2008) [3] PHYSICAL REVIEW A 81(3) 033819 (2010)
※ This research was conducted by Ono under the supervision of Professor Holger F. Hofmann at the Graduate School of Advanced Sciences of Matter, Hiroshima University.
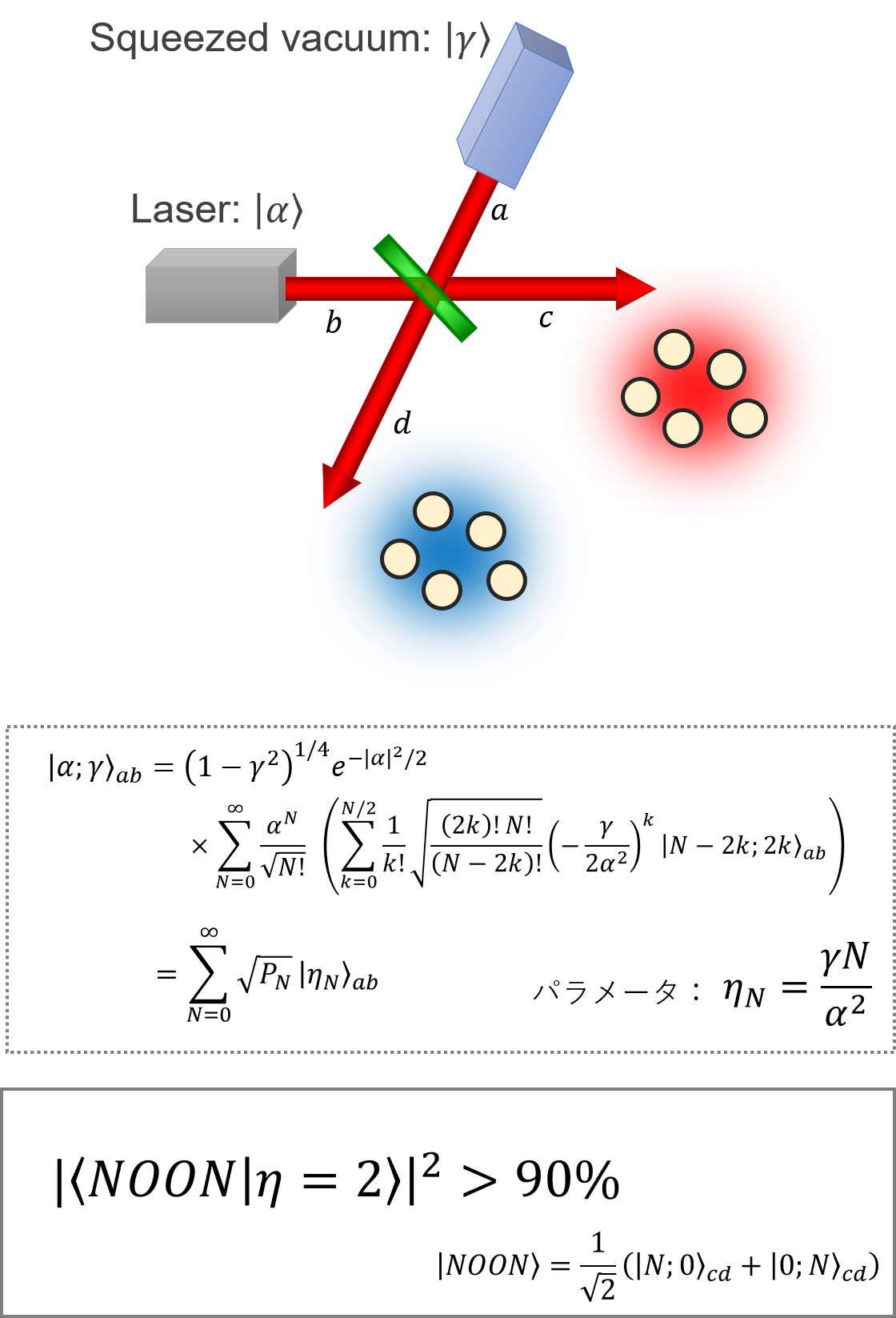
An Entanglement-Enhanced Microscope
In this study, we successfully developed the world’s first quantum entanglement microscope by integrating entangled photons into a microscopy system.
Entangled photons exhibit unique properties that cannot be explained by classical mechanics. When used in measurement, they enable sensing with a sensitivity beyond the standard quantum limit, which is unattainable with any classical light source.
Among optical microscopes, differential interference contrast (DIC) microscopes utilize the principles of interferometric spectroscopy, enabling the observation of unstained specimens that are not visible under standard transmitted light microscopy. However, until now, the measurement sensitivity of DIC microscopes has been fundamentally constrained by the standard quantum limit.
In this study, we successfully developed the world’s first quantum entanglement microscope by applying quantum measurement techniques with entangled photons to differential interference contrast (DIC) microscopy, achieving sensitivity beyond the standard quantum limit. Furthermore, using the developed microscope, we visualized a glass substrate with a step height of approximately 17 nm and achieved an observation with a signal-to-noise ratio (SNR) about 1.35 times higher than that of a conventional microscope.
[1] Nature Communications 4(2426) (2013)
※ This research was conducted by Ono in Professor Shigeki Takeuchi’s laboratory at Hokkaido University (currently at the Graduate School of Engineering, Kyoto University).
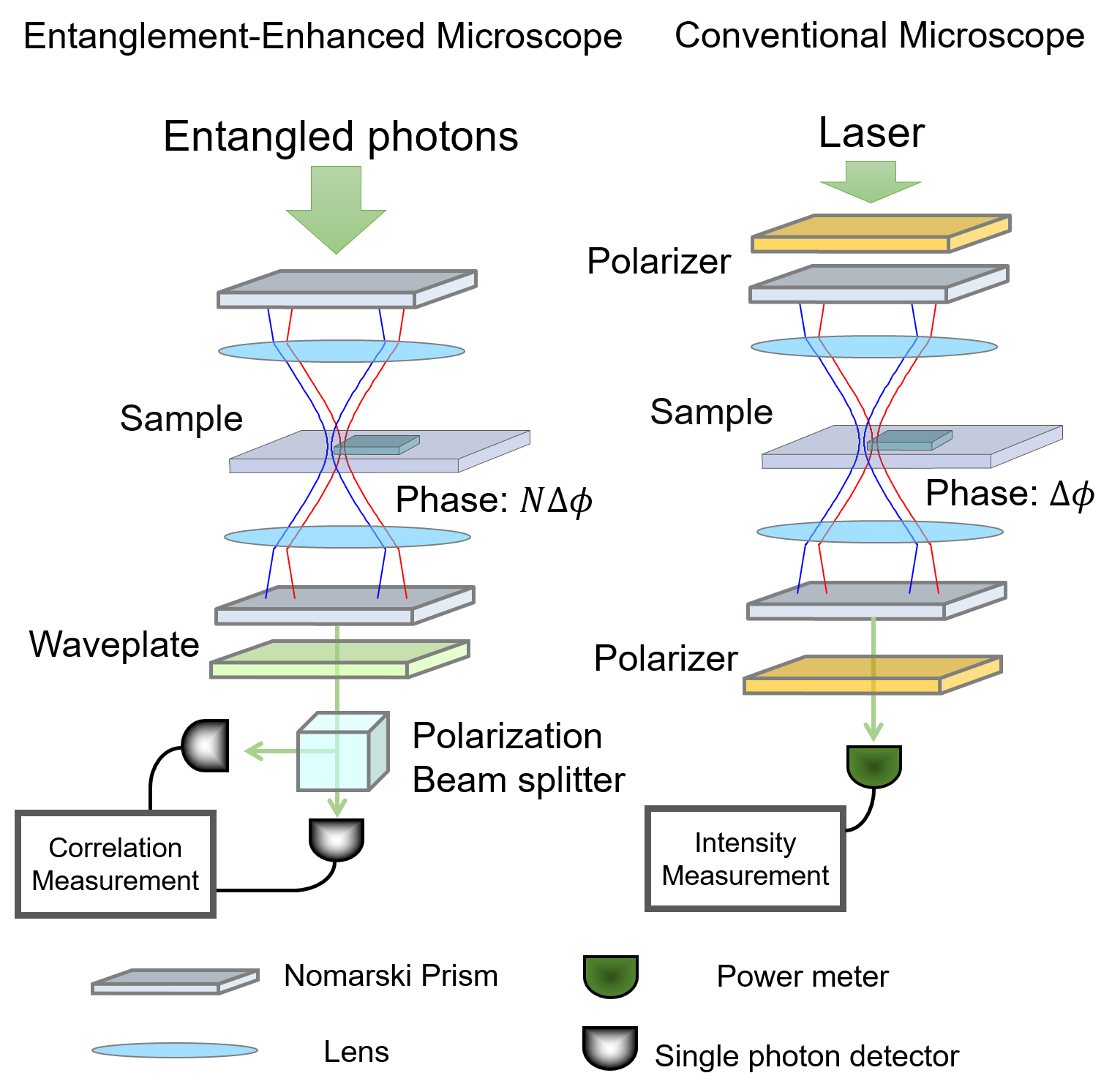
Optical Spin-Squeezed State
In this study, we successfully generated an optical spin-squeezed state in which five photons are quantum mechanically entangled. Furthermore, by utilizing this quantum state as a probe for optical phase measurement, we achieved phase estimation with a sensitivity exceeding the standard quantum limit.
Entangled photons exhibit fluctuation properties that differ from those of classical light. By precisely controlling and leveraging these fluctuations, it is possible to measure a target’s characteristics with greater sensitivity than classical light sources, such as lasers.
In this study, we employed the parametric process in a nonlinear optical crystal to generate six photons and then extracted one photon to artificially induce and control quantum fluctuations. Furthermore, using the generated optical spin-squeezed state, we measured the optical phase difference and achieved a precision approximately 1.34 times higher than that of conventional classical light-based methods.
This study is expected to contribute to the advancement of quantum measurement with sensitivity beyond the classical limit and to a deeper understanding of multi-particle quantum entanglement, driving progress in a wide range of fields, from fundamental science to practical applications.
[1] NEW JOURNAL OF PHYSICS 19 053005 (2017)
※ This research was conducted by Ono in Professor Jeremy O’Brien's laboratory at the University of Bristol, UK.
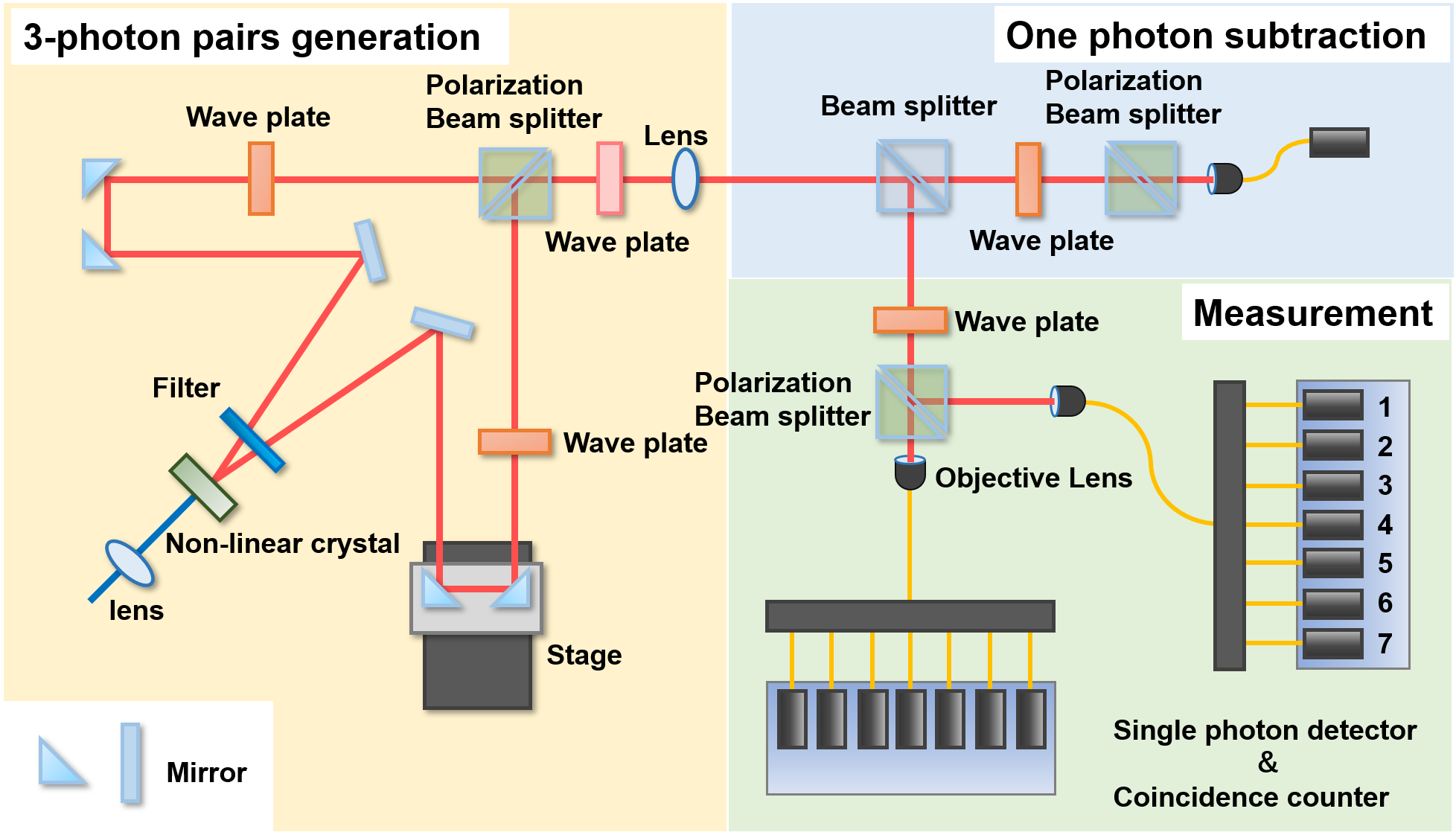