Research
Motivation
Photonic integrated circuits are expected to serve as a platform for realizing large-scale optical devices. Among them, silicon-based photonic integrated circuits offer several advantages, including high integration density, advanced fabrication techniques, and compatibility with telecommunication wavelengths and CMOS technology. These advantages make silicon photonic integrated circuits a promising platform for quantum information processing.
In our laboratory, we utilize well-established silicon photonics technology to develop photonic integrated circuits that enable advanced control of quantum states of light for applications in computation and measurement. Specifically, we harness nonlinear optical effects to generate entangled photons on silicon waveguides, manipulate quantum states using optical and electrical circuits, and accurately detect individual photons with single-photon detectors. Our goal is to advance large-scale quantum photonic technologies.
Generation of Entangled Photons on Silicon Waveguides
Entangled photon pairs exhibit unique properties that cannot be explained by classical mechanics. Leveraging these properties allows for measurements that surpass classical limits and computations based on entirely different principles from conventional methods.
In this research, we generate entangled photon pairs on-chip by leveraging the nonlinear optical effects of silicon. Specifically, we employ spontaneous four-wave mixing (SFWM) in silicon to convert two pump photons into signal and idler photons, thereby creating entangled photon pairs (Figure 1).
Figure 2 shows an example of the entangled photon pair generation rate as a function of pump light intensity. Our group utilizes these entangled photon pairs as input states for optical quantum circuits and explores various applications.
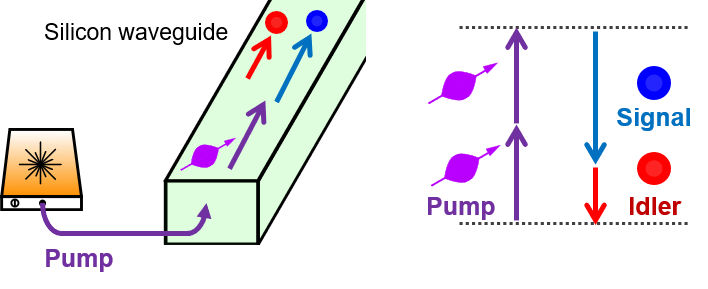
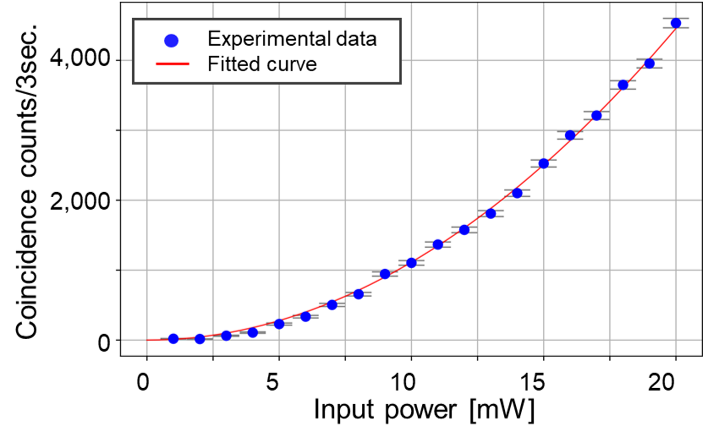
Design of Optical Quantum Circuits
By leveraging silicon photonics technology, we can implement optical quantum circuits that allow for advanced control of quantum states of light.
In this research, we design optical components to control quantum states of light by applying principles of electromagnetism and computer simulations.
Figure 1 illustrates a filter designed to separate entangled photons from laser light. To achieve this separation, we use an asymmetric Mach-Zehnder interferometer with different path lengths (Figure 1a). Evaluation of the designed filter demonstrated an extinction ratio exceeding 30 dB (Figure 1b). By integrating these components and performing optical quantum operations, we aim to explore novel quantum phenomena and develop new quantum technologies.
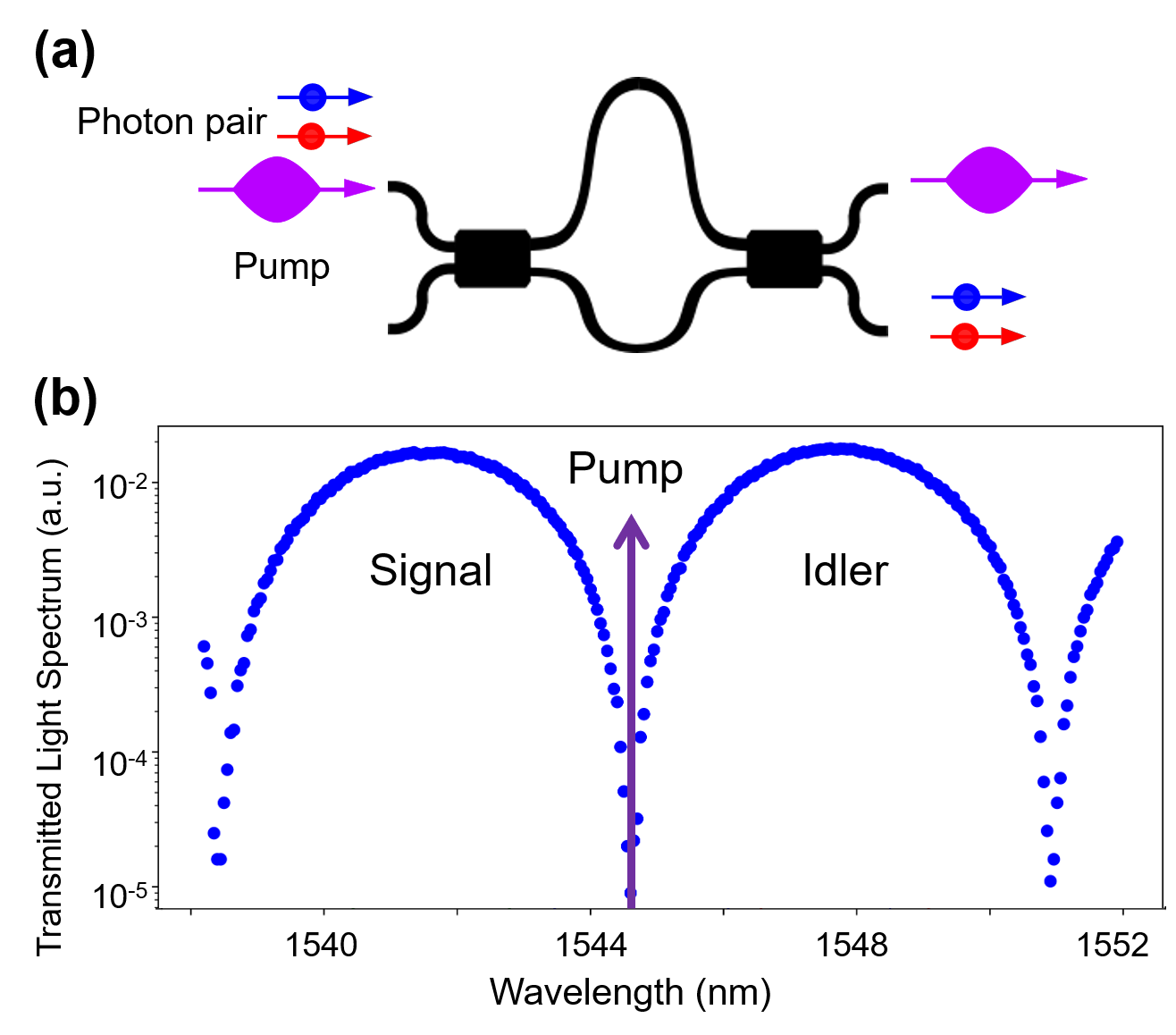
Implementation of a Nonlinear Interferometer
By actively leveraging nonlinear optical effects within optical quantum circuits, we can perform more advanced and highly functional quantum operations than ever before. While previous studies have demonstrated nonlinear interferometers using bulk optical systems, their implementation on photonic integrated circuits had not been reported until now (Figure 1).
In this research, we leveraged our expertise in quantum silicon photonics to integrate quantum entangled photon generation, interferometers, and filters onto a silicon substrate, successfully implementing the world’s first nonlinear interferometer on a photonic integrated circuit (Figure 1). Furthermore, evaluation of the implemented nonlinear interferometer demonstrated a quantum interference visibility of approximately 97% (Figure 2).
This achievement highlights the potential of nonlinear interferometers as a fundamental building block for large-scale optical quantum circuits.
[1] Optics Letters 44(5) 1277 - 1280, March 2019 [2] Laser Research 48(9) 499 - 504, September 2020
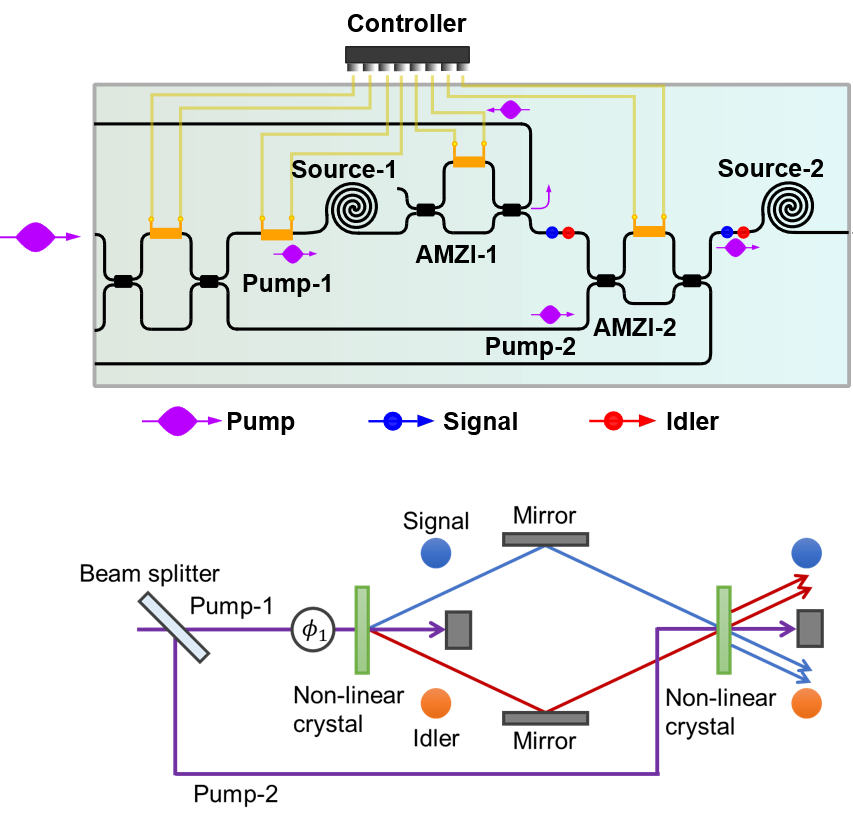
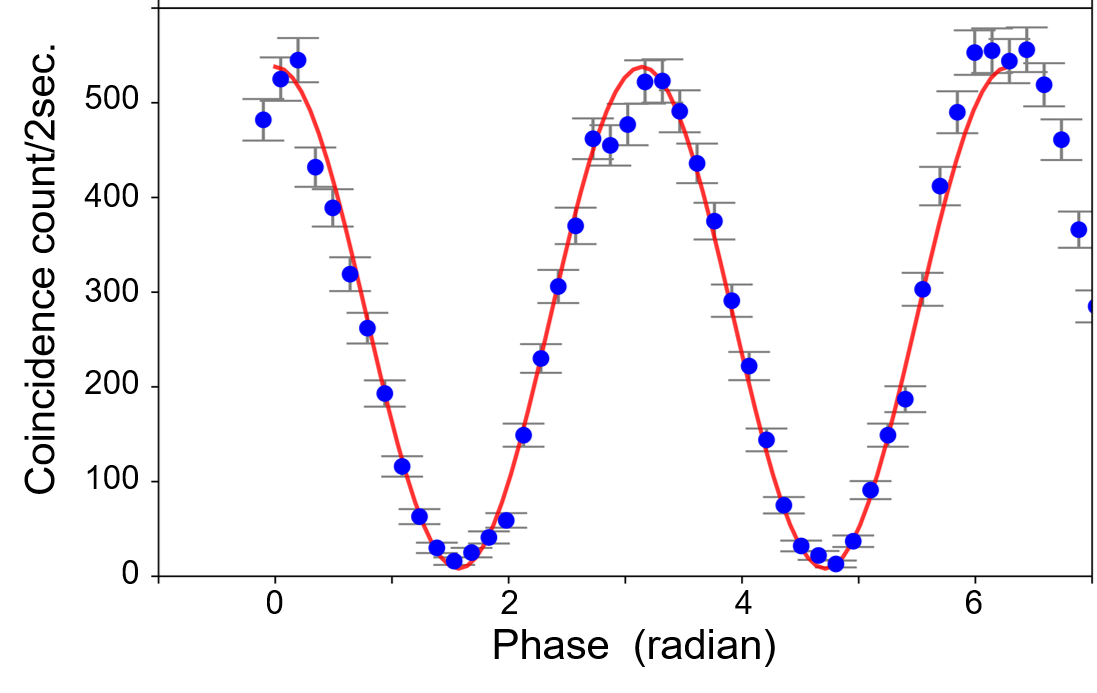
Research Before the Establishment of the Laboratory
"Research conducted before the establishment of the laboratory can be found" here